TABLE OF CONTENTS
Catecholamines
3,4-dihydroxybenzene is called catechol; therefore, drugs that contain this structure are called catecholamines.
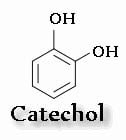
Epinephrine, norepinephrine, and dopamine are known as endogenous catecholamines, while isoproterenol is a synthetic catecholamine.
Epinephrine (EPI), norepinephrine (NE), and isoproterenol (ISO) exhibit varying agonistic actions on adrenoceptors.
The alpha-adrenergic effect of catecholamines (contraction of smooth muscle) follows the order:
EPI ≥ NE >> ISO
The beta-adrenergic effect (relaxation of smooth muscle) follows the order:
ISO > EPI
Effects of Catecholamines
* | Norepinephrine | Epinephrine | Isoproterenol |
Alpha effects | Potent alpha stimulant (usually less than EPI) | Most potent alpha stimulant | least potent |
Beta effects | Weak stimulant of blood vessel β2 receptor | Potent β stimulant | Most potent β stimulant |
Synthesis of Catecholamines
The precursor for catecholamine synthesis is tyrosine.
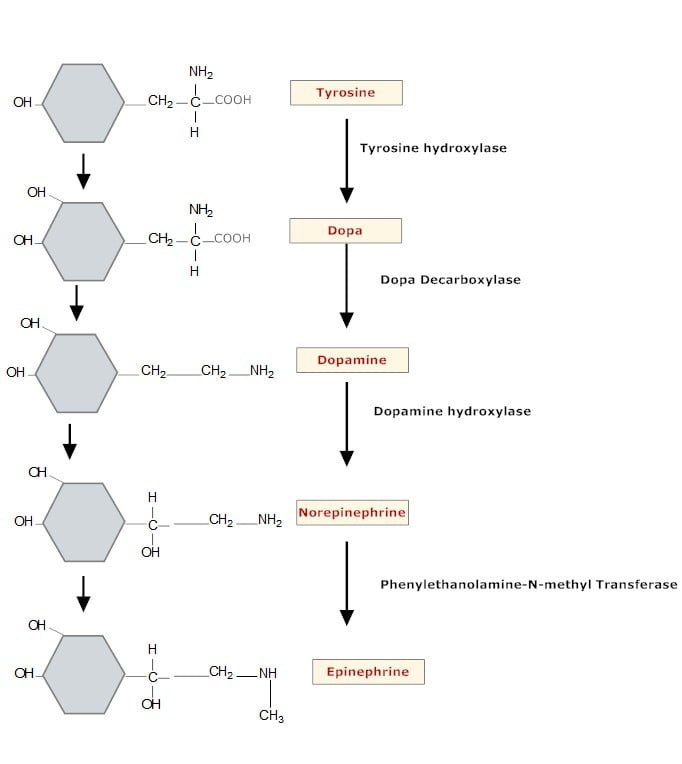
Conversion of phenylalanine to tyrosine takes place in the liver, while the conversion of tyrosine to DOPA, and DOPA to dopamine, occurs in the cytoplasm of adrenergic neurons.
Dopamine is converted to norepinephrine within granules, and norepinephrine is converted to epinephrine in the adrenal medulla.
Tyrosine hydroxylase is the rate-limiting enzyme, and its inhibition by alpha-methyl-ρ-tyrosine results in depletion of catecholamines. All enzymes involved in catecholamine synthesis are relatively non-specific and can act on closely related substrates. Tyrosine hydroxylase is activated by cAMP-dependent protein kinases and inhibited by catecholamines.
Storage within the granular vesicles is achieved by complexation of catecholamines with adenosine triphosphate (ATP) and a specific protein called chromogranin. This complex renders the amines inactive until released. The vesicles also contain ascorbic acid and dopamine β-hydroxylase.
Catecholamines are taken up from the cytoplasm into the granules via an active transport system that is ATP- and Mg++-dependent. This intragranular pool of norepinephrine is believed to be the primary source of neurotransmitter release during nerve stimulation.
Release from the storage vesicles occurs via calcium-dependent exocytosis, which is induced by depolarization of the nerve ending. Certain drugs can also induce release by destroying storage vesicles or displacing catecholamines from the vesicles.
Amines in the cytoplasm may either be taken up by granules for storage or be inactivated by monoamine oxidase (MAO), a deaminating enzyme located in the neuronal mitochondria. Intracytoplasmic dopamine may also be deaminated by MAO.
Norepinephrine that has been taken back into the nerve terminal may either be restored in granules or deaminated by MAO. Reuptake is an active, energy-dependent mechanism. Enzymatic conversion accounts for about 20% of norepinephrine inactivation, while reuptake is the major mechanism for terminating its action. The initial inactivation involves two enzymes: Monoamine oxidase (MAO) and Catechol-O-methyltransferase (COMT).
Catecholamines present in the blood are metabolized in the liver by COMT and MAO. Aldehyde reductase and aldehyde dehydrogenase further metabolize the aldehydes produced by MAO-mediated deamination. Aldehyde reductase catalyzes the formation of alcohol products, while aldehyde dehydrogenase catalyzes the formation of acid products.
Products from one enzyme can serve as substrates for another. The major final products are:
- 3-methoxy-4-hydroxyphenylethylene glycol (MOPEG)
- 3-methyl-4-hydroxymandelic acid (VMA)
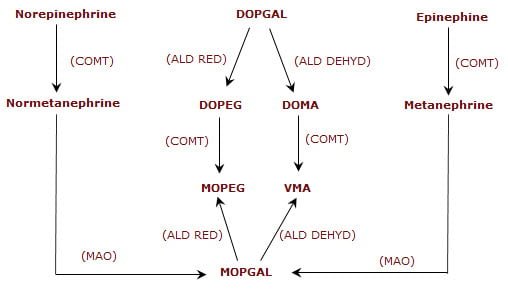
Structure Activity Relationship
- Maximum sympathomimetic activity is observed when two carbon atoms separate the aromatic ring from the amine group.
- Increased bulkiness of substitution on the nitrogen atom (N-atom) enhances beta receptor activity.
- The lesser the substitution on the N-atom, the greater the overall activity.
- Removal of one or both hydroxyl (OH-) groups reduces β-receptor activity.
- Substitution of the ring hydroxyl group decreases potency.
- Beta-carbon side chain substitution results in less active central effects.
- Alpha-carbon substitution yields a compound not susceptible to monoamine oxidase (MAO).
- Alkyl substitution on the amino group affects both alpha and beta agonist properties.
Mechanisms of Action
Catecholamine hormones act in the same way as catecholamine neurotransmitters, i.e., they bind to α- and β-adrenoceptors on the target cell membrane.
Noradrenaline (norepinephrine) is more effective than adrenaline (epinephrine) at α-receptors, while adrenaline (epinephrine) is the more potent agonist at β-receptors. Activation of these receptors modulates the intracellular concentrations of a variety of second messengers; for example, levels of cAMP may rise or fall.
The pattern of second messenger response actually observed varies from cell to cell and depends on the receptor subtype involved. These intracellular signals alter cell function, often through second messenger-dependent kinases, which phosphorylate important functional proteins and thereby alter their biochemical activities.
Pharmacological Actions
1. Excitatory
- Blood vessels – constriction (including veins, increased venous return to the heart).
- Iris – contraction of the radial muscle with mydriasis.
- GI & Urinary – contraction of the sphincters.
- Sweat glands – secretion in horses.
- Salivary glands – viscous secretion.
- Male genitalia – ejaculation.
2. Inhibitory
- Bronchioles – dilatation.
- Blood vessels – dilatation.
- GI Tract – relaxation of smooth muscle.
- Urinary bladder – relaxation of the detrusor.
3. Cardio-excitatory Effects
- SA node – increased heart rate.
- Atria & Ventricles – increased force of contraction and conduction velocity.
- AV node & His-Purkinje system – increased automaticity and conduction velocity.
4. Metabolic Effects
- Liver – glycogenolysis and gluconeogenesis, leading to hyperglycemia.
- Pancreas – decreased (α) and increased (β) secretion.
- Fat cells – lipolysis, increased plasma free fatty acids.
- Skeletal muscle – glycogenolysis, increased blood lactate.
Therapeutic Uses
- Acute anaphylactic reaction – To counter hypotension (α) and bronchospasm (β), epinephrine is the drug of choice.
- Allergic disorders, asthma – The objective is to produce bronchodilation via β2 receptors. Isoproterenol or epinephrine can be used by inhalation or by intramuscular or intravenous injection. They are short-acting and also produce marked cardiac stimulation via the β1 receptors. Ephedrine can also be used. Though it is long-acting, it produces marked CNS stimulation.
- Cardiac arrest/heart block – Intracardiac injections of epinephrine or isoproterenol, followed by IV infusion or subcutaneous and intramuscular injections, are useful.
- In combination with local anaesthetics – To produce local vasoconstriction and retard the removal of the anaesthetic, thereby increasing the duration of anaesthetic action. Epinephrine is preferred for this use.
- Control of bleeding – Epinephrine, when applied locally, arrests bleeding from arterioles and capillaries.
- Decongestion of mucous membrane – Phenylephrine and pseudoephedrine are used in rhinitis, sinusitis, and hay fever as decongestants.
- Ophthalmology – Ephedrine or phenylephrine can be used to examine the eye, as they induce mydriasis without cycloplegia.
- Shock – Use of α agonists to maintain blood pressure in shock may be harmful by reducing perfusion to the kidneys and brain, which are already affected. However, dopamine is useful in the treatment of cardiogenic, traumatic, and hypovolemic shock because it selectively dilates the kidney blood vessels, increasing glomerular filtration and urine production. Additionally, dopamine increases blood supply to abdominal organs.
- Hypertension – Clonidine is effective in hypertension, and its effect is primarily central. It may partially activate peripheral presynaptic α2 receptors.
- Uterine relaxants – Isoxuprine can be used to produce uterine relaxation (tocolytic).